Magnetic Nozzles
Research supported by:
National Defense Science and Engineering Graduate Fellowship, and
the Princeton Plasma Physics Laboratory's Program in Plasma Science and Technology
Introduction
Simply stated, a magnetic nozzle converts thermal energy of a plasma into directed kinetic energy. This conversion is achieved using a magnetic field contoured similarly to the solid walls of a conventional nozzle (see, for example, Fig. 1). The applied magnetic field in most cases possesses cylindrical symmetry and is formed using permanent magnets or electromagnetic coils, which confines the plasma and acts as an effective “magnetic wall” through which the thermal plasma expands into vacuum. Applications include laboratory simulations of space plasmas, surface processing, and plasma propulsion for spaceflight.
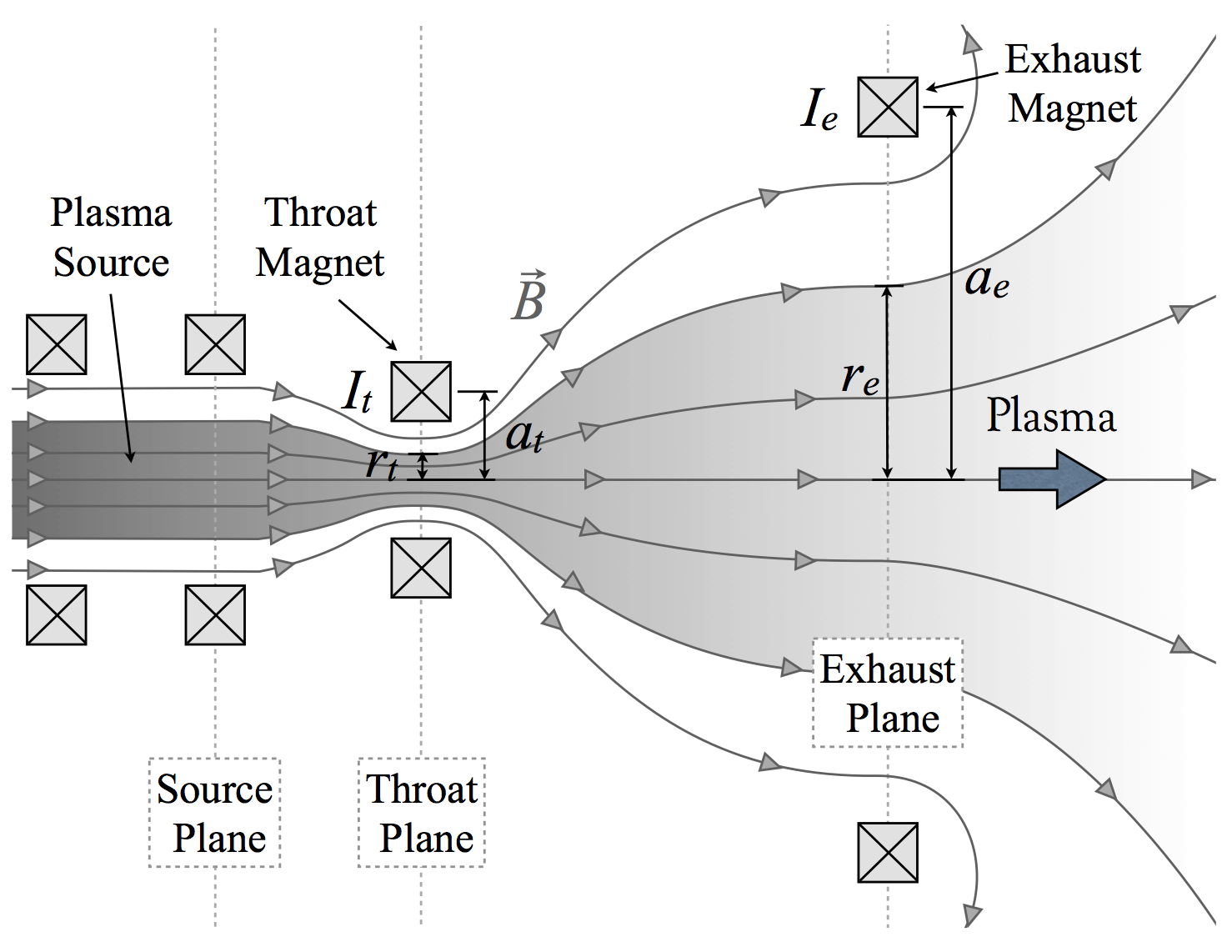
Magnetic nozzle research at the EPPDyL began in 2008. The goal of this research is to understand the fundamental physical processes of the plasma flow through the nozzle and their impact on the nozzle performance. Specifically, we are working towards answering the following important questions:
- How do the dynamics of the plasma flow through the magnetic nozzle influence the exhaust plume structure?
- How is the exhausted plasma affected by the relationship between the physical and magnetic goemetries?
- How does the magnetized plasma detach from the applied magnetic field?
These questions will be answered through a combination of theoretical, experimental, and computational research. Ultimately, knowledge of these processes will yield fundamental scaling laws for the performance of magnetic nozzles for plasma propulsion.
Theory
We are developing theoretical models to study the relationship between the plasma dynamics in the magnetic nozzle and the structure of the exhaust plume. The structure of the exhaust plume is important to the overall propulsion performance of the nozzle. A highly divergent plume delivers less thrust than a plume that is well-directed. Furthermore, the exhaust plume structure is intimately tied to the physical mechanisms by which the plasma may detach from the magnetic field of the nozzle.
The nature of magnetic nozzle plasma expansion has been previously observed, both experimentally and with numerical models, to develop significant spatial non-uniformities in the exhaust plume. Motivated by these observations, we have used a transformation from cylindrical to magnetic coordinates (Fig. 2) to derive the first analytical model capable of providing closed-form solutions for the two-dimensional distributions of the plasma potential, density, and ion Mach number in the expansion region of the nozzle (Fig. 3).


Our model ultimately yields fully analytical expressions for the beam divergence efficiency and thrust coefficient a magnetic nozzle. It is found that these performance parameters depend mainly on the ion Mach number, plasma radius, and density profile uniformity at the nozzle exhaust plane. The performance of the nozzle is most sensitive to the plasma radius, with improved performance observed for a decreasing radius due to the lower effective divergence of the applied magnetic field.
Experiment
The Magnetic Nozzle Plasma Dynamics and Detachment eXperiment (MN-PD2X) was designed to experimentally study the fundamental plasma physics associated with plasma flow through a magnetic nozzle. The goals of the experiment are to:
- Characterize the relationship between the plasma source and magnetic field geometries and its effect on plasma transport and wall losses.
- Determine how these factors influence the plasma properties at the nozzle throat.
- Determine how the plume structure changes with respect to the plasma flow at the nozzle throat and compare the results to our the theoretical model.
The foundation of the experiment is a flat-coil radio frequency (RF) plasma source designed to operate up to 1 kW. An L-type matching network is used to match the RF source and antenna impedances. The plasma source is mounted concentrically with two electromagnetic (EM) coils, each with a peak magnetic field of 500 G.
The MN-PD2X was designed to allow easy modification of the plasma source aspect ratio (ratio of the source tube length to radius) and magnetic field geometries to test the effects of the relationship between the physical surfaces of the plasma source and the magnetic field topology. Specifically, the location of the rear wall of the plasma source may be adjusted relative to the exhaust plane, thus altering the aspect ratio. Furthermore, the topology of the magnetic field may be changed by adjusting the relative locations of the EM coils (Fig. 4).

The plasma source is mounted on a swinging-gate style thrust arm within the EPPDyL’s Large Dielectric Pulsed Propulsion (LDPP) Facility. To allow thrust stand measurements, the source tube is physically isolated from the antenna and matching network.
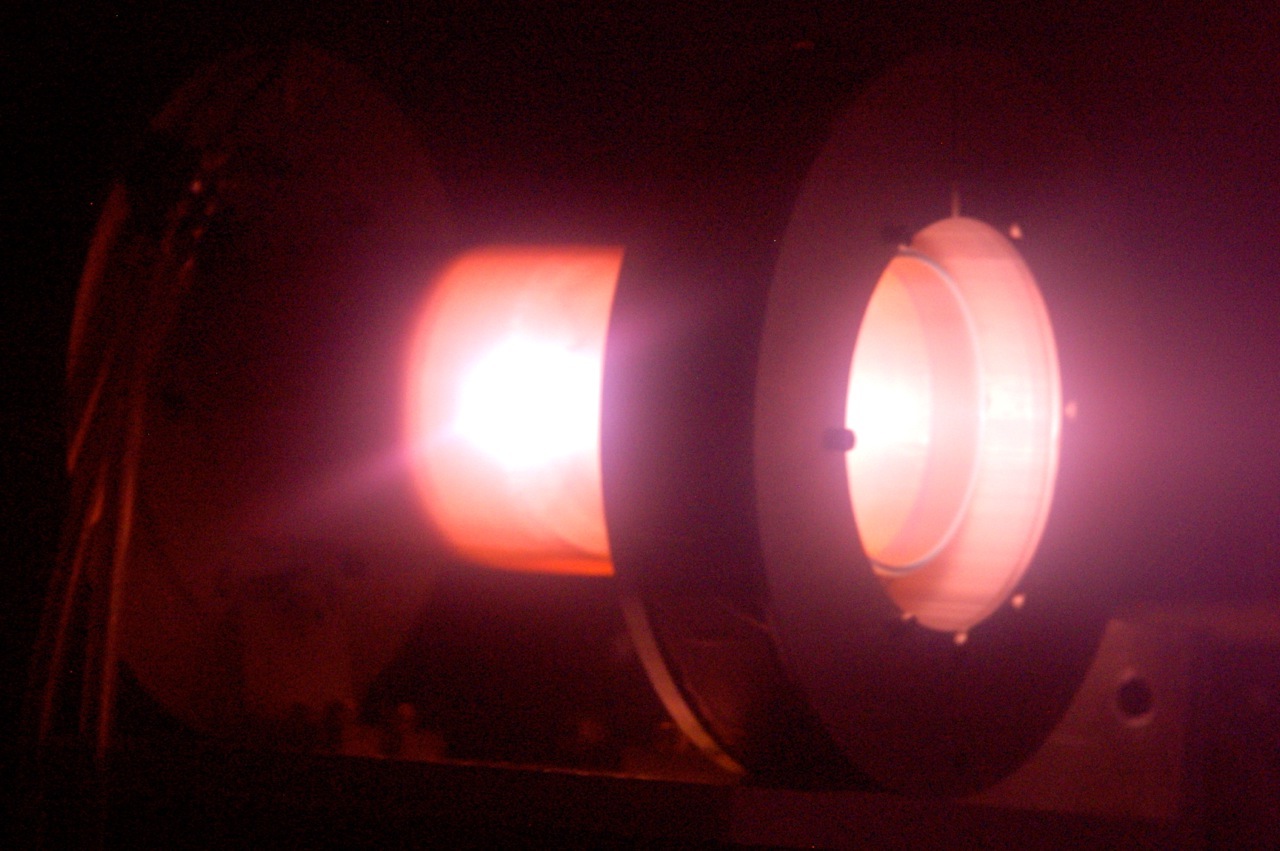
A photograph of the first MN-PD2X plasma may be seen in Fig. 5. The discharge was obtained at 250 W with an Argon gas mass flow rate of approximately 1 mg/s and a peak field of 250 G. The location of the adjustable rear wall may be seen about halfway between the two EM coils. The absence of a parasitic discharge behind the rear wall indicates a good seal between the antenna and plasma source chamber.
Computation
In collaboration with Professor Sam Cohen and Dr. Adam Sefkow
The mechanism by which plasma detaches from the applied magnetic field of the nozzle remains poorly understood. There are, however, many promising theories. Unfortunately, these theories involve complex plasma processes that are difficult to include within self-consistent analytical models. Furthermore, the plasma conditions under which these processes become dominant are difficult to characterize experimentally with conventional diagnostics. To this end, we are working towards applying the particle-in-cell code, LSP, to the problem of magnetic nozzle plasma detachment. Using LSP, we hope to validate pre-existing detachment theories and potentially discover new and relevant physics.
Relevant Publications
- Critical Condition for Plasma Confinement in the Source of a Magnetic Nozzle Flow - IEEE Transactions on Plasma Science (2015)
- Performance Scaling of Magnetic Nozzles for Electric Propulsion - Ph.D. Dissertation (2015)
- Propulsive Performance of a Finite Temperature Plasma Flow in a Magnetic Nozzle with Applied Azimuthal Current - Physics of Plasmas (2014)
- Influence of the Applied Magnetic Field Strength on Flow Collimation in Magnetic Nozzles - 50th AIAA/ASME/SAE/ASEE Joint Propulsion Conference (2014)
- Experimental Verification of Plasma Focusing by Azimuthal Current in a Magnetic Nozzle - 50th AIAA/ASME/SAE/ASEE Joint Propulsion Conference (2014)
- Thrust and efficiency model for electron-driven magnetic nozzles - Physics of Plasmas (2013)
- A Novel Thrust Measurement Method For Plasma Rockets with Magnetic Nozzles Using B-field Measurements - B.Sc. Thesis (2012)
- Plasma detachment and momentum transfer in magnetic nozzles - 47th AIAA/ASME/SAE/ASEE Joint Propulsion Conference and Exhibit (2011)
- Similarity Parameter Evolution within a Magnetic Nozzle with Applications to Laboratory Plasmas - 31st International Electric Propulsion Conference (2011)
- The Influence of Induced Currents on Magnetic Nozzle Acceleration and Plasma Detachment - 46th AIAA/ASME/SAE/ASEE Joint Propulsion Conference (2010)
- Divergence of a Propulsive Plasma Flow Expanding through a Magnetic Nozzle - 31st International Electric Propulsion Conference (2009)
Contact
Former students:- Justin Little
- Margaret Shaw
- Lorenzo Ferrario
- Jaume Navarro Cavallé